Medical devices that can be broken down and excreted by the body could reduce the number of surgeries needed to remove implantable sensors and stimulation devices.
Implantable medical technologies are often engineered for durability. For example, pacemakers, which help the heart beat at a normal rate, are left functioning in the body for up to seven years.
But what if we want to implanted technologies to last for shorter periods of time? In that case, biodegradable devices would be ideal, says Lan Yin, a biomedical scientist from Tsinghua University’s School of Material Science and Engineering, based in Beijing.
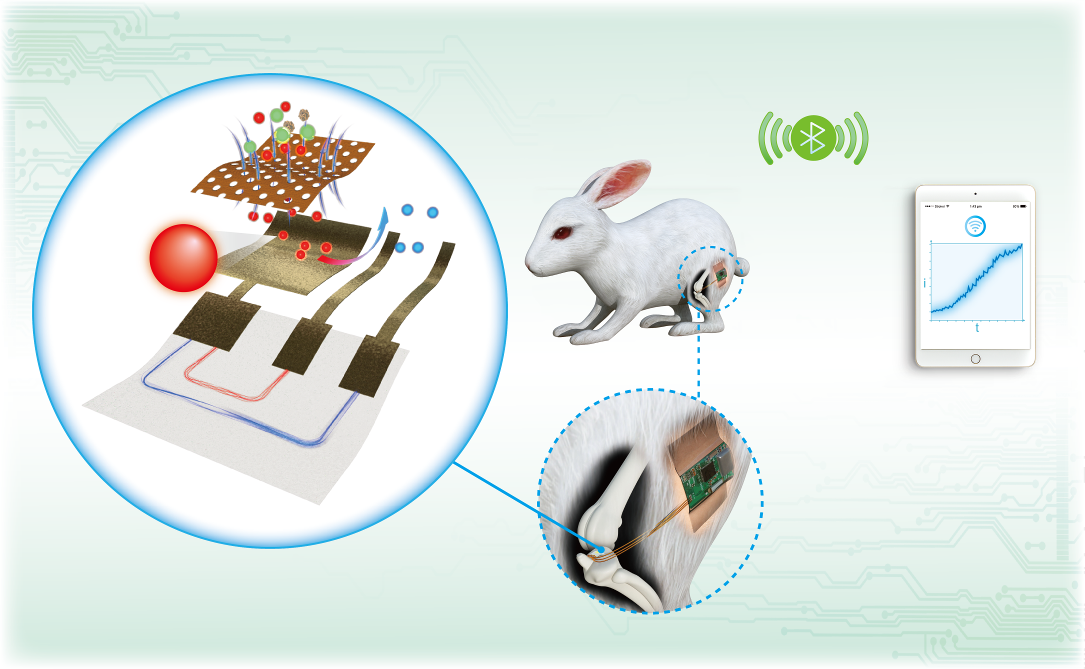
In 2020, Lan Yin’s team published on their fully biodegradable, highly sensitive nitric oxide sensor. The sensor was shown to be capable of continuous detection of nitric oxide in the joint cavities of rabbits and wireless data transmission for up to five days. The team used their device to demonstrate that high levels of nitric oxide are linked to the injection of pro-inflammatory cytokines into the joint.
Yin leads a team that is working on non-toxic device components that could safely break down and dissolve over time, ranging from batteries to entire nerve regeneration stimulators.
“Most of our devices are designed to be fully degradable — able to be completely metabolized and excreted from the body,” Yin explains. If implant can be removed from the body in this way, she points out, it could eliminate the need for additional surgeries to retrieve the devices, reducing infection risks, recovery time and hospital costs.
The proposed devices will be tiny, weighing just a few milligrams or micrograms. “That way the amount of material introduced to the body is minimal, which further ensures biocompatibility,” she says.
Biodegradable parts
Making fully biodegradable devices is not as easy task. Each component goes through a very different degradation process and must be safely cleared by the body, Yin explains. For example, device surfaces, which are often made from polymers, can sometimes be broken down by water, degrading to small molecules.
The metallic components and semiconducting materials used in batteries can also be dissolved in aqueous solutions and result in ions that can be excreted by the body, says Yin.
Other materials are metabolized through phagocytosis, the process a cell uses to break down unwanted pathogens or cellular materials. For this to happen, a cell’s plasma membrane engulfs a large particle and then destroys it using cytotoxic oxidants, peptides and degradative enzymes.
For safe, biodegradable devices to be realized, a number of these strategies must come together so the materials break down at a rate that aligns with their needs for treatment, Yin explains. This will be no mean feat.
Nonetheless, she currently sees peripheral nerve regeneration as a potential early application of biodegradable technology. Roughly 3% of all hospital trauma injury intake is thought to features some peripheral never damage, she says — almost half do not fully recover. Severe injuries can result in the loss of sensory and/or motor function.
Microsurgical interventions are first-line treatments in such cases, but delivering electrical signals to damaged nerves has been shown to offer cues that promote the nerve regeneration, greatly reducing recovery times in both animal and clinical studies.
“It has become a very promising non-pharmacological therapy to aid nerve regeneration,” says Yin. The electrical stimulation appears to encourage the extension of dendrite or axon protrusions — the parts of the nerve that send and receive signals to and from other parts of the nervous system.
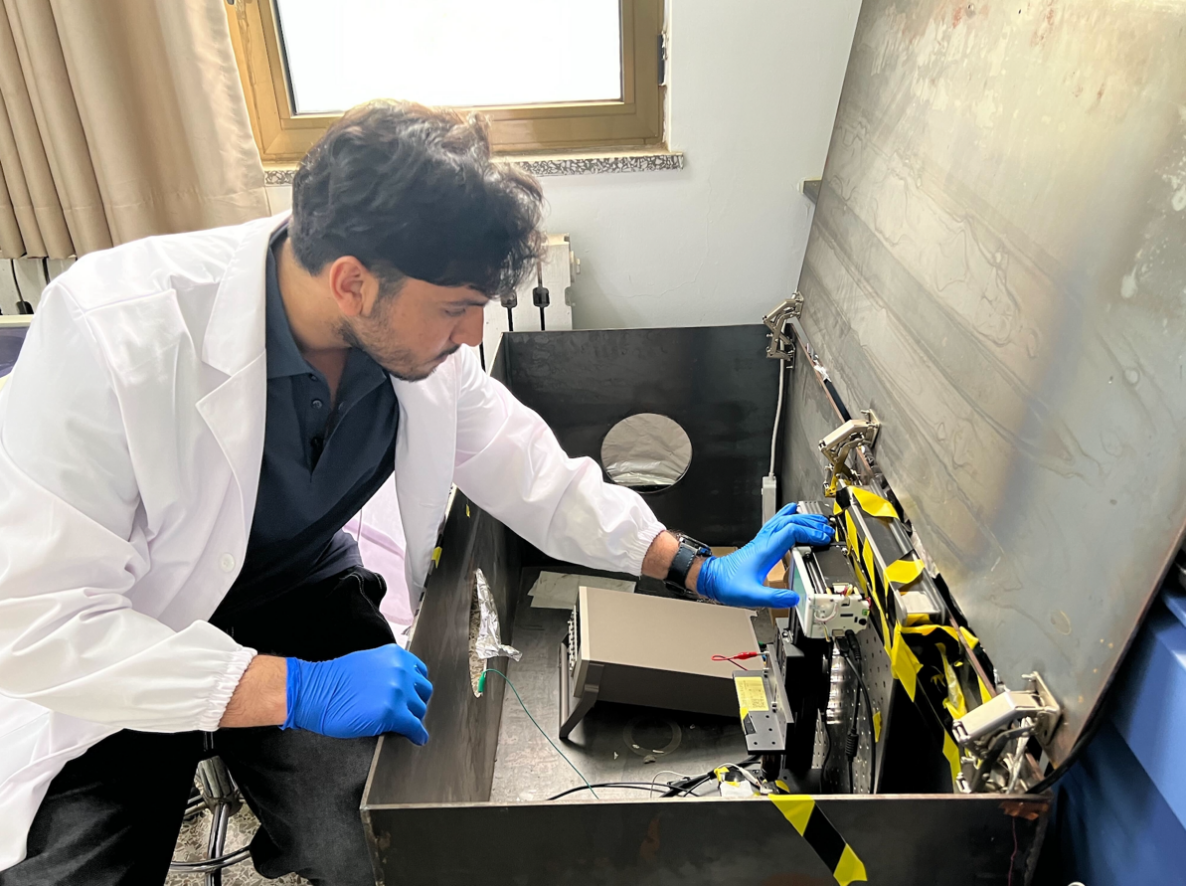
A member of Lan Yin’s team, Abdul Aziz, checks a device wirelessly receiving data from an implanted, biodegradable sensor.
Stimulating nerve regeneration
The development of a fully biodegradable, self-powered, implantable electrical stimulation device would enable the delivery of continuous electrical cues without the need for additional surgeries for removal, adds Yin.
In 2022, her team reported their creation of a biodegradable, self-electrified, and ultra-miniaturized electrical stimulation device for exactly this type of scenario.
Using their device, her team accelerated the recovery of rodent motor function following a cut that left a 10 mm gap in the test animal’s sciatic nerve — the nerve that travels from the lower spine, down the back of each leg to the foot. The device also has a functional lifetime of up to three days and would likely fully degrade after 56 days under the accelerated conditions in the human body, the researchers found1.
The technology has comparable features to non-biodegradable products that are already commercially available for different uses during surgeries, says Yin. The team now hopes to make a version of their device that can function for a longer period.
To do this, Yin’s team are tackling a particularly tricky aspect of the technology — biodegradable batteries with prolonged lifetimes. It’s challenging to keep producing enough power as a battery degrades, explains Yin.
In 2023, her team came up with a zinc-molybdenum battery that had a functional lifetime of 19 days. They then tested it in a stimulation device designed to promote the growth of ganglia on nerves that that send and receive signals to other parts of the nervous system, and which produce gaseous signalling molecules that modulate the behaviour of cells2.
Typically, biodegradable batteries last from a few days to a few weeks, says Yin, so this development is promising. The researchers are now testing the efficacy of zinc-molybdenum batteries for encouraging nerve regeneration in rodents.
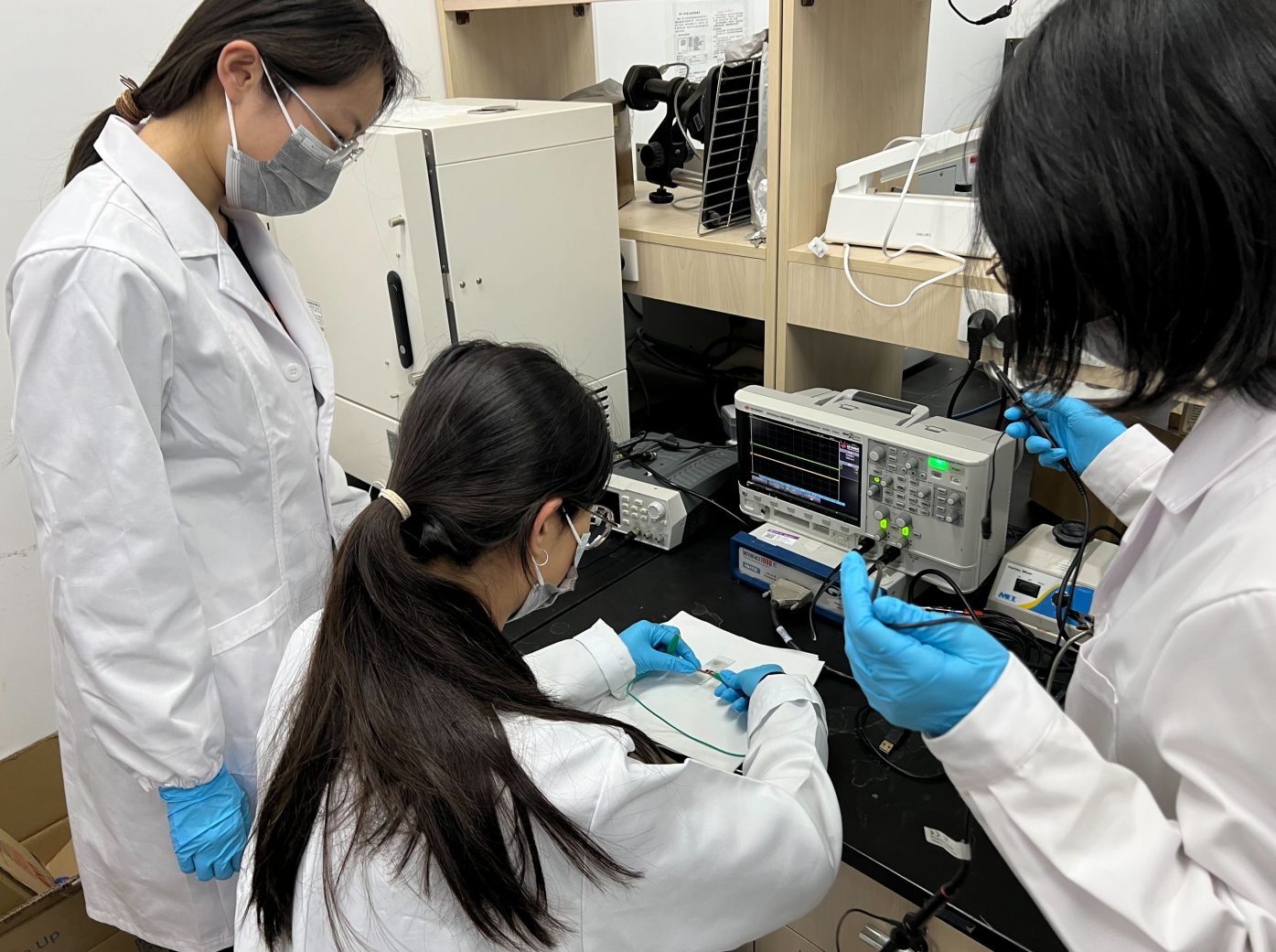
Researchers at Tsinghua University’s School of Material Science and Engineering check their biodegradable batteries.
Ephemeral sensors
There are still many challenges to tackle before usable biodegradable devices can be realized. Fabrication must also be scalable, and the devices must be thoroughly tested in clinical trials.
Nonetheless, the possibilities are exciting. Beyond uses for regenerating tissues such as nerves, Yin sees biodegradable devices having promise as electrochemical sensors.
In 2020, her group published a paper on a biodegradable sensor3 designed to monitor abnormal levels of nitric oxides in the joint cavities of rabbits.
Not only did the team achieve a fully biodegradable, highly sensitive nitric oxide sensor capable of wireless data transmission and continuous detection of the gas for up to five days, their results also suggested that a high level of nitric oxide is linked to the injection of pro-inflammatory cytokines into the joint.
A follow-up study revealed that high levels of nitric oxides in the joint after anterior cruciate ligament (ACL) ruptures can be linked to the onset of osteoarthritis — a degenerative joint disease — later in life. “The development of a biodegradable nitric oxide sensor offers an essential strategy to probe nitric-oxide related diseases,” Yin says.
Low-impact sensors like this, that can track a person’s health data, could become commonplace, as personalized medicine takes off, Yin adds. “Considering rapid advancements in human-machine interfaces, biodegradable neural interfaces could emerge as another possibility for this technology too,” she says.
Neural interfaces create a direct communication pathway between the brain’s electrical activity and an external device, most commonly a computer or robotic limb. The computer or robotic can then be controlled directly via a person’s thoughts. In this context, biodegradable neural interfaces might be used to help patients operate intermediary mobility or communication aids during stroke rehabilitation, for example, says Yin.
It's an exciting time for biotechnologies, says Yin. In the next few years, she and her team expect to continue to develop a diverse array of biodegradable components that could find their way into devices. She has high hopes that a biodegradable nerve regeneration device could reach clinical trials in that time too.
References
1. Wang, L., Lu, C., Yang, S., Sun. P., Wang, Y. et al. Sci. Adv. 6, eabc6686 (2020). doi:10.1126/sciadv.abc6686
2. Huang, X., Hou, H., Yu, B., Bai, J., Guan, Y. et al. ACS Nano 17(6), 5727–5739 (2023). doi: 10.1021/acsnano.2c12125
3. Li, R., Qi, H., Ma, Y., Deng, Y., Liu, S. et al. Nat. Commun. 11, 3207 (2020). doi: 10.1038/s41467-020-17008-8
Editor: Guo Lili